It’s happened to nearly everyone. You go to use a simple electrical device…a flashlight, a voltmeter, a TV remote, or a child’s toy…and the battery is dead. It has become exhausted and must be replaced. Or worse, you open the device to find that the battery has corroded, possibly damaging or even destroying the contacts. In some critical respects, essentially the same thing can happen to the largest standby battery system that might be providing backup support for a substation, a computer room, a hospital, a manufacturing process — anywhere uninterruptible power is essential.
Standby battery banks can corrode, short out, lose capacity through unintentional grounds or aging, and fall victim to a host of other breakdowns and failures. They can also be victim to an out-of-sight, out-of-mind mentality. Most electrical functions are readily observable: The lights are on, motors are running, the building is heated. But standby batteries are tucked away out of sight and often forgotten. That is, until there’s an event.
When a utility experiences a fault, the battery bank should immediately come on line and provide alternate power until the utility comes back on line. Failure to do so can be nothing short of catastrophic. Switchgear can fail to operate to open faults. Buildings can catch fire, production lines stall and sacrifice materials, computer rooms go off line and lose data, and hospital patients on life support could die.
To prevent such tragedies, standby battery banks must be tested regularly for their continued ability to come on line and deliver full power whenever utility service is interrupted.
TESTING BATTERY STRINGS
A battery string is tested with a battery impedance tester that includes a DC current source that injects a test current through the string, then measures the voltage drop and calculates the impedance. An array of problems, including terminal corrosion, plate sulphation and corrosion, dry-out, and many more can increase the impedance to inhibit or prevent current propagation and take the battery out of operation.
Many battery banks are arranged in tiers of parallel strings. This can increase the supplied current, capacity, or reliability of the strings. Parallel strings allow the current delivery capability to be doubled. They also increase capacity, allowing the load to be supported for a longer period.
Flooded lead-acid batteries, which are usually used in series strings, can fail in a shorted mode. They are not technically shorted and will still allow current to pass through them. They just aren’t making a contribution to the output, but the string still remains in service.
Sealed lead acid batteries (VRLA), which are typically used in parallel strings, tend to fail in open mode. They are not literally open, but current will not readily pass through them. Failure of one battery will stop current propagation in that string, but the parallel strings will still permit current flow and maintain the backup function. Lithium ion batteries use a management system that monitors safety parameters and will open the battery in case of a hazard. Parallel strings are recommended. If one cell opens, current can still pass through the other string(s).
Accordingly, parallel strings have notable advantages in overcoming failed cells and still being able to provide critical backup power to avert emergencies. But their arrangement introduces a fundamental testing complication. When testing a series string, test current has only one path — through the string. But when testing a parallel string, test current has multiple paths depending on the number of strings.
A battery tester measures the total current it is able to inject, but it looks at the voltage drop between the potential probes. In a series string, current has a single path and remains constant, giving an accurate measurement of impedance across the cell (Figure 1).
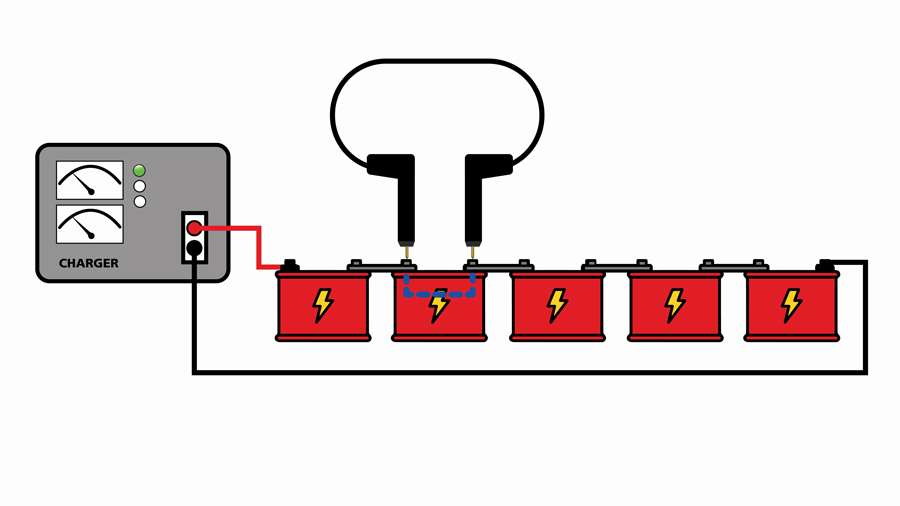
Figure 1: Series String
In a parallel string, current is divided between the strings. The impedance tester measures the total current through the string but only the voltage drop between the potential probes, thereby calculating an inaccurate measurement of cell impedance based on more current than is actually traveling through the tested cell (Figure 2). A bad cell can therefore appear acceptable.
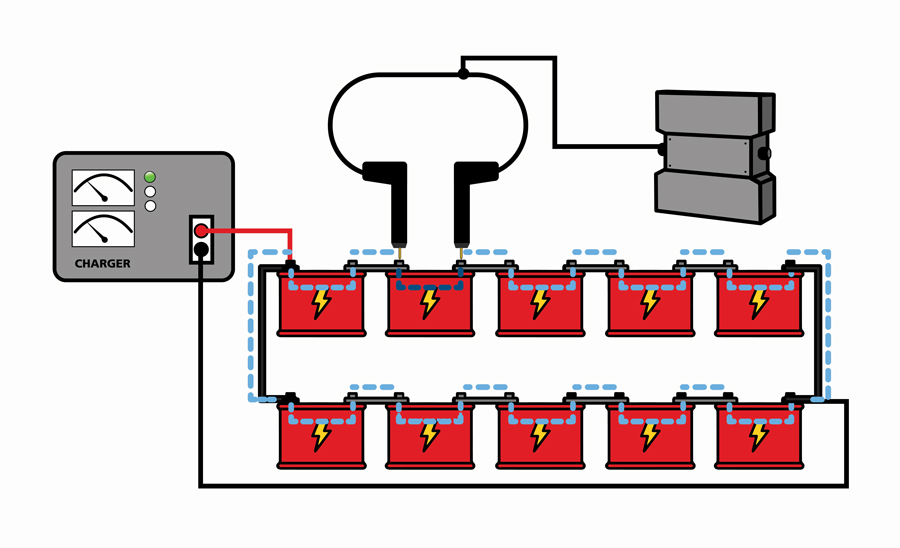
Figure 2: Parallel String
As an example, suppose the battery consisted of two tiers of five cells, each with 100 mΩ impedance (Figure 3). The charger is applying half an amp. The impedance tester measures 0.0375 V across an individual cell. Since the string is balanced, only 0.25 A is flowing through the tested cell. The true measurement for this cell’s impedance would be 150 mΩ. However, the impedance tester measures the total current propagating through the entire string, which is 0.5 A. It calculates and displays 0.75 mΩ — half the actual value. It is obvious that a deteriorated cell could test good under these circumstances, potentially rendering the string off line if called into service.
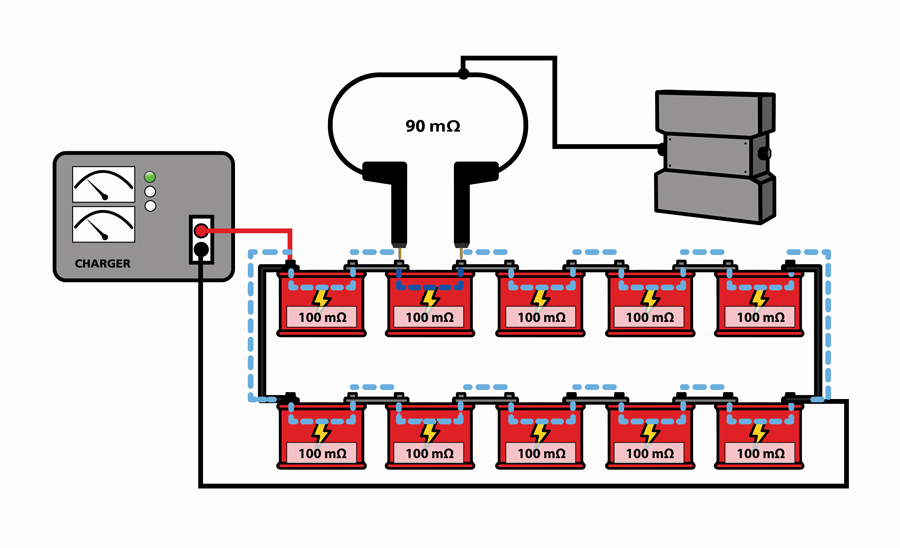
Figure 3: Parallel String Sample Calculation
STANDARDS & TECHNOLOGY
Traditionally, this issue was addressed by IEEE Std. 1188, Recommended Practice for Maintenance, Testing, and Replacement of Valve-Regulated Lead-Acid (VRLA) Batteries for Stationary Applications. This standard calls for taking the string off line and segmenting the parallel strings. This is a lot of work! Fortunately, modern technological improvements have produced a convenient methodology that allows the testing and troubleshooting of parallel strings without segmentation and without taking them off line.
The technological breakthrough that augments this process is the split-core current clamp, which appeared in the mid- to late-20th century. The current clamp can intercept and measure escape current that is propagating through the parallel strings, and the measurement circuit in the impedance tester can deduct this from the calculation. The impedance of the specific cell being tested is now calculated against the specific current passing through it and not the total current being injected by the impedance tester (Figure 4). The measurement is correct for the specific cell being tested, and faulty high-impedance cells will not elude detection.
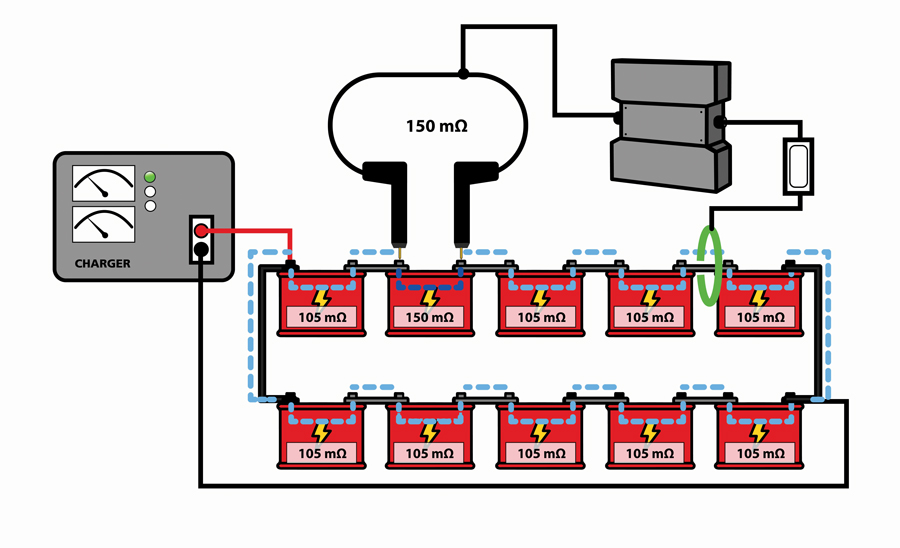
Figure 4: Parallel String with Current Clamp
BATTERY FAILURE
Routine preventive maintenance of standby batteries is easy to bypass because they aren’t visibly active on a daily basis like computers, lighting, and machinery. But neglecting maintenance can come at a high price and all at once in the form of catastrophic and potentially lethal failure.
Internal chemical decomposition can emit gases that cause the battery to swell and explode, releasing dangerous fumes. Failure of backup power to utility circuit breakers and relays can allow fault currents to wreak enormous damage. VRLA batteries can emit hydrogen as part of their normal operation. If left unattended in a poorly ventilated, poorly temperature-regulated battery room, hydrogen gas can accumulate and ignite, producing an explosion and massive structural damage. And although not physically destructive or threatening, failure of backup power to a computer room can result in a crippling loss of vital data.
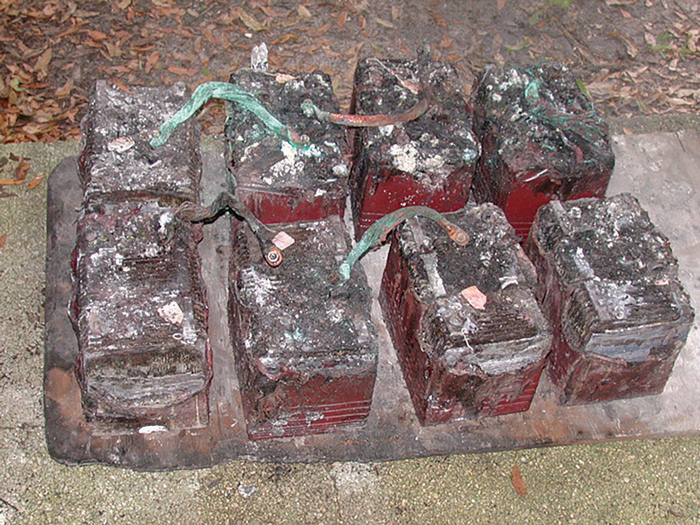
Battery Destroyed by Thermal Runaway
Finally, National Electric Reliability Council (NERC) requirements have become mandatory and enforceable for all bulk power system owners, users, and operators in the United States. Founded in 1968 in response to devastating blackouts, NERC requirements for stationary battery backup strings require battery terminal connection resistance and intercell connection resistance tests to be performed and documented.
CONCLUSION
Modern technology has produced instruments that make the seemingly daunting task of testing the readiness and reliability of large, multi-tiered battery banks an integral part of your preventive/predictive maintenance program. This can be accomplished without taking the system off line. Data analysis and storage software enables complete and detailed record keeping for effective continuity of the program and presentation for NERC inspection. A comparatively small investment of time and effort in a maintenance program with a full-quality battery impedance tester can prevent and save the cost of prohibitive damage and outages in the electrical system.
Andrew Sagl is the Power Quality and Battery Testing Product Manager at Megger. He has been with Megger for 20 years and is a specialist in power quality and battery testing technology and application. Andy develops and supports power quality equipment in addition to writing power quality and battery publications and delivering training and seminar courses. He has a degree in electronics and is a member of the IEEE Power Engineering Society and Battery Standards Group. In the past, Andy has specialized in nano-motor technology as well as military sub-systems and weapons guidance systems.