The design life of a transformer is usually about 20 to 25 years. In the United States, estimates show the average age of a transformer is currently around 42 years, which means a number of even older units are still in service. As with all things, transformers age and degrade over time. Transformer failures can come from a variety of sources including through faults, faulty crimps and brazes, corrosive sulfur, poor design, water intrusion, collateral damage from a bushing or load tap changer (LTC) failure, incorrectly sized leads, and a host of other issues. Overall, however, the demise of a transformer from normal aging is linked directly to the condition of the solid insulation (i.e. paper, cellulose).
PAPER INSULATION
Paper is used as an insulating medium because it is inexpensive, a very effective dielectric, has high mechanical strength, and allows insulating liquids to fill voids in the insulating structure. Much of the mechanical strength of paper and pressboard comes from the long-chain cellulose polymer. As the cellulose ages, the polymers are cleaved and become shorter, resulting in reduced mechanical strength.
Although the two main insulation components in a transformer are the insulating liquid and the cellulose (paper), it is the paper that is the main driver of transformer life, as it is intimately wrapped around the conductor and cannot be easily replaced, nor can it be remediated in situ. In contrast, the insulating liquid can easily be replaced, reclaimed, or reconditioned.
However, the same stressors that negatively impact the insulating liquid will also impact the paper. The main stressors to insulating liquids are heat and oxygen. Minor stressors are organic acids and possibly the catalytic effects of copper. Water has little to no effect on the life of the insulating liquid. Paper degradation is accelerated by heat and oxygen, as are insulating liquids, but the added stressor of water is significant and is directly proportional to the water concentration in the paper. Also like insulating liquids, the minor stressors of organic acids and metal catalytic effects play a role. Insulating liquids and paper degradation has been discussed in numerous papers over the years and thus will not be discussed in detail in this article.
ASSESSING PAPER INSULATION CONDITION
Since paper degrades, it becomes vitally important to monitor the degradation process and take effective measures to reduce or delay the aging process. Several analytical tools can be used to assess the condition of the paper insulation. These tools are broken down into two large categories: direct and indirect tests.
Direct Tests
These tests are conducted directly on the paper insulation from the transformer. The two most common tests are tensile strength and degree of polymerization (DPv). Both tests require paper samples from the transformer. The DPv test measures the average length of the cellulose molecule in the paper. DPv values of 1,000 to 1,300 are considered consistent with new paper; as values of 200 would be considered end-of-life, and values of 400 would be considered midlife as it is a logarithmic relationship as opposed to linear. McNutt proposed a life curve based on the DPv value.
Taking a paper sample for DPv or tensile strength is an expensive proposition. Some or all of the insulating liquid must be drained from the transformer and samples dissected from various locations in order to adequately assess the aging of the insulation. Then those sections where samples were retrieved must be repaired followed by preparing the transformer to receive and install the insulating liquid back into the unit.
The biggest obstacle is deciding where in the transformer to retrieve the paper sample. An attempt needs to be made to find the hottest spot insulation as this will dictate the survivability of the transformer over time. Most often, the hottest spot insulation is in the upper third of the winding and cannot be retrieved unless the transformer is scrapped. Paper aging in transformers is not uniform; it follows thermal and water gradients. In addition, external layers are more exposed to higher concentrations of oxygen and the byproducts of aging in the insulating liquid. Actual DP measurements from various areas of the transformer can be drastically different depending on the local conditions.
Because of the sheer intrusiveness and exorbitant cost, this is seldom done on in-service transformers. Additionally, if it is a generating unit, revenue is lost as a result of the transformer being out of service while the process in ongoing. Although direct tests can provide information on the paper in the general vicinity from which they were taken, it cannot provide a global determination of the transformer insulation as a whole. This is why there has been a focus on indirect tests to gather valuable information.
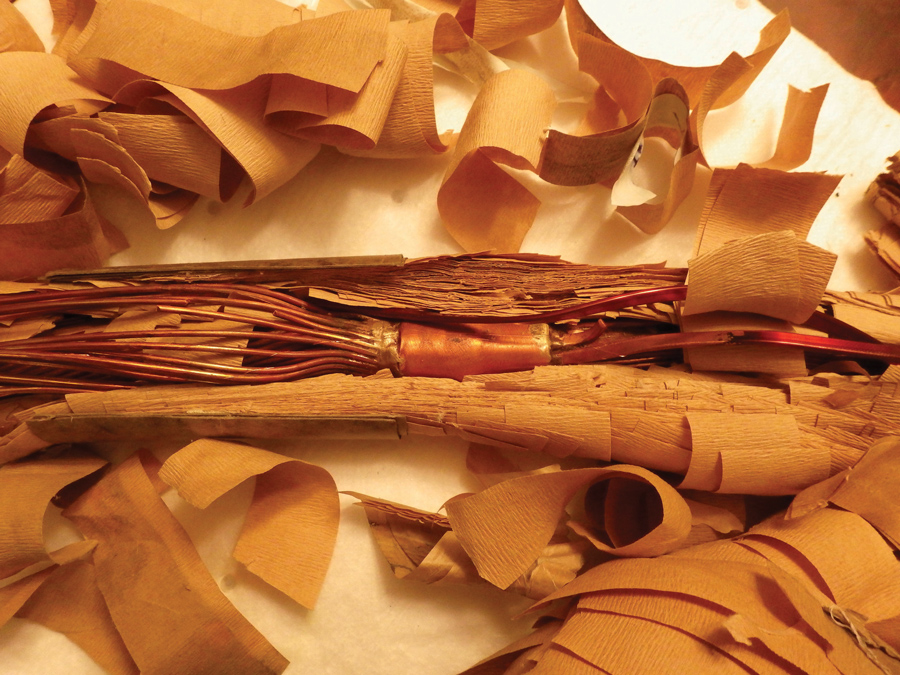
Figure 1: Example of the Amount of Paper around a Lead
Indirect Tests
Most indirect tests are the analysis of chemical markers in the insulating liquid. Electrical tests such as power factor, dielectric frequency response (DFR), gas-in-oil monitors, and water-in-oil monitors can help one understand the condition of the cellulose, but the results of these tests are unable to define the aging process relative to how much of the cellulose life has been consumed.
Indicators of cellulosic aging are:
- Water content/relative saturation
- Acidity of the oil
- Carbon oxide concentrations
- Furanic compounds
- Methanol and ethanol concentrations
Water Content
Analyzing the water content of the oil using on-line monitors or water-in-oil measurements can provide some indication of the condition of the paper. Along with DFR, they can provide information on the water content of the paper through mathematical interpretation. As the water content of the cellulose doubles, the life of the paper decreases by half, so it is directly proportional. However, determining the end of life of the paper or how much life has been consumed through these means is not effective.
Acid Neutralization Number
The acid content (neutralization number) is another marker that paper has decayed as the broken bonds in the cellulose structure will eventually form measurable acids. But other materials in the transformer also form acids. Natural esters in the insulating liquid, for example, forms large amounts of acids. Therefore, it becomes difficult to distinguish between paper degradation and other materials being degraded. So, although useful in a general sense, this test has not been used to determine the remaining life of paper insulation.
Carbon Oxide Gases
Carbon oxides — carbon monoxide (CO) and carbon dioxide (CO2) — can be detected from dissolved gas-in-oil (DGA) or online DGA sensors that alert the user that paper degradation has occurred. Carbon oxides can be produced from outgassing of transformer components, especially in older transformers, but generally most of the production is from cellulose decay. There have been attempts to use carbon oxide concentrations to estimate paper life, especially in Japan (Yoshida, et al), but it is not common practice. It is probably more suitable to sealed transformers that are not leaking as opposed to free-breathing transformers that can lose much of the CO to the atmosphere because of the partitioning coefficient.
Furanic Compounds
Furanic compounds are probably the most notable of the cellulose degradation indicators that began to develop as a method of analysis in the 1980s in Europe.
Through the hydrolytic, oxidative, and thermal stressors already discussed, the polysaccharide of cellulose (Figure 2) is broken down into smaller and smaller molecules forming a variety of products including alcohols, carbon oxides, waters, acids, and free glucose that is not soluble in the insulating liquid. To form furanic compounds, the glucose undergoes additional reactions that break the six-membered carbon rings into five-membered ring structures (Figure 3). Unsworth and Mitchell demonstrated a mechanism by which the open-chain glucose molecule goes through a series of dehydration reactions (elimination of water molecules) and then recycles into a five-membered ring structure. Furanic compounds, unlike sugars such as glucose, are oil soluble and therefore are detectable.
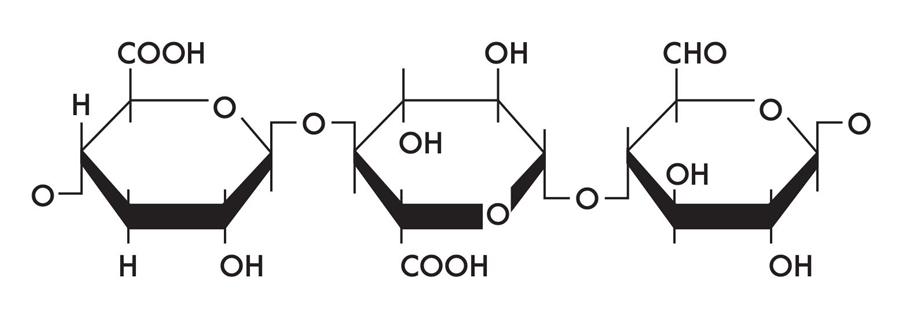
Figure 2: Three Units of a Cellulose Molecule
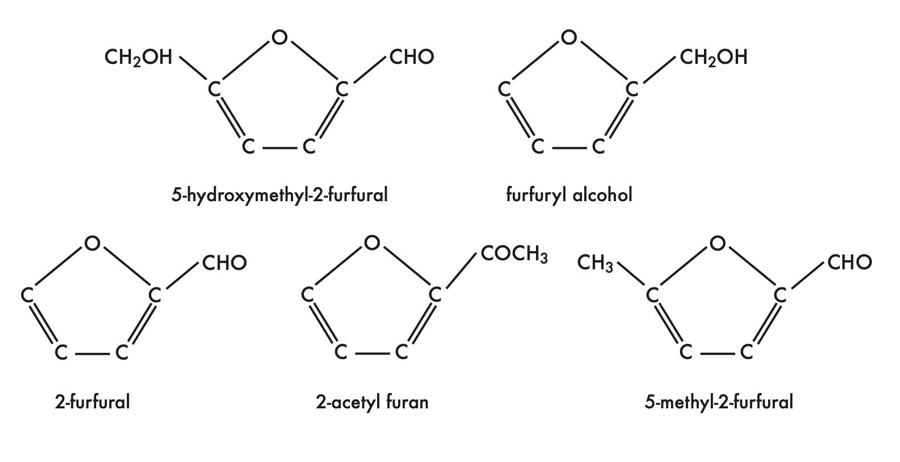
Figure 3: Commonly Found Furanic Compounds
ANALYZING FURANIC COMPOUNDS
ASTM Method D5837 and IEC 61198 are the methods employed to perform furanic compound analysis; they can be utilized for all insulating liquids including natural and synthetic esters. The method involves extracting semi-polar compounds, in which furanic compounds might be present, from the insulating liquid into a more polar solvent that is then injected into a high-performance liquid chromatograph (HPLC). In the HPLC, the extracted eluent is passed through an analytical column that separates the compounds by polarity and molecular weight. The compounds are detected by the use of a tunable ultra-violet (UV) detector optimized at specific wavelengths for the desired compounds. Furanic compounds are measured in ug/L or mg/kg. Although there are many furanic compounds, many of them are unstable in the insulating liquid or the eluent and are of little use for routine diagnostic information.
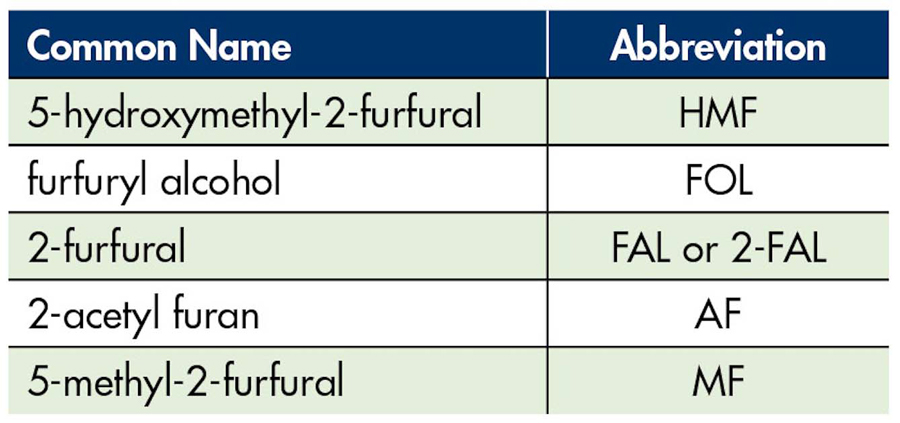
Table 1: Furanic Compounds Commonly Used for Diagnostic Purposes
One compound, 2-furfural (FAL or 2-FAL), is the one most commonly present and used for diagnostic purposes. The 2-furfrual content is being used to provide an overall DPv value through the use of the Chendong or other similar equations.
Although a powerful tool for transformer diagnostics, it can be misapplied, as it was originally designed for free-breathing Chinese-made Kraft paper transformers, even though it is now used universally for all transformers. The equation is given as:
Equation 1: Estimated DP =
((log of 2-furfural in mg/kg) – 1.5)/-0.0035
The furanic compounds produced from locations of paper degradation inside a transformer are comingled from other locations in a transformer to provide a pseudo-average 2-furfural concentration in the oil, much like water and gas-in-oil measurements. The extent to which paper is aged in one or more locations will increase the value of the 2-furfural concentration.
The DPv value calculated by the Chendong equation does not apply to any one location within the transformer. It must be remembered that if the 2-furfural concentration is high, which corresponds to a low DPv value, the actual location in the transformer where the highest rate of paper degradation is taking place is probably much worse.
As stated, the specific population of transformers the Chendong equation is derived from were Kraft paper-wound, free-breathing, conservator-type transformers. Varied practices were used worldwide to manufacture transformers. Many transformers in Latin America, Europe, and parts of Asia, for example, have historically been manufactured with breathing conservator systems and Kraft paper insulation. Most open conservator systems seal out water, but not oxygen. In contrast, the most common practice in the United States is to seal the oil and paper from air by using either a sealed tank with nitrogen or a conservator tank with a polymer membrane. For older U.S. designs with a 55°C average winding-temperature rise, Kraft paper insulation was typically used before the 1960s. Most modern U.S. designs are of the 65°C average winding-temperature-rise type, and thermally-upgraded (TU) paper is used to wrap conductors. In some cases, especially in mobile transformers, Nomex© insulation is employed; Nomex is a synthetic aramide fiber that does not produce furanic compounds.
The Chendong equation has come to be used by some to evaluate the paper DP for all transformers, regardless of paper type or preservation system. As transformers with different insulation and preservation systems accumulate different amounts and ratios of furanic compounds, they will have differing aging profiles. The results of the calculation to estimate DP from the 2-furfural content needs to consider the kind of paper and the type of preservation system. For example, when used for nitrogen-blanketed and sealed-conservator transformers, this calculation can seriously underestimate the degree of long-term aging, especially in transformers with thermally upgraded insulation. Under these conditions, the result could provide a false sense of security.
The Chendong equation is best used to estimate an average DP in transformers with Kraft paper insulation and free-breathing conservators. For other insulation and preservation systems, the equation can be used to estimate paper degradation from thermal events.
Because of the differences in paper types and preservations systems, there are four categories for evaluating the results (Table 2). In addition, normal rates of accumulation concentrations of 2-furfural are provided for each category.
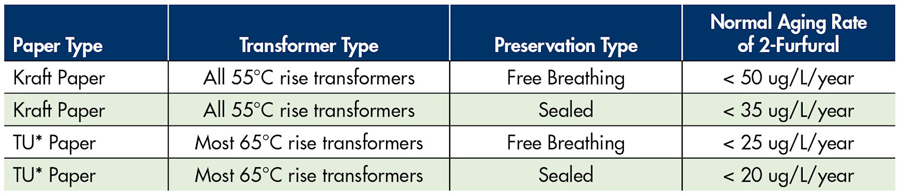
Table 2: Categories of Transformer Types
These rates can be calculated over the age of the transformer or from sample to sample. It is actually good to perform both calculations, as the current aging may be more severe than previous aging rates and is dependent on load increases over time, overheating events, environmental conditions, and leaks in sealed systems (Figure 4). To get a sense of severity and the degree of remaining life, the information in Table 3 may be useful.
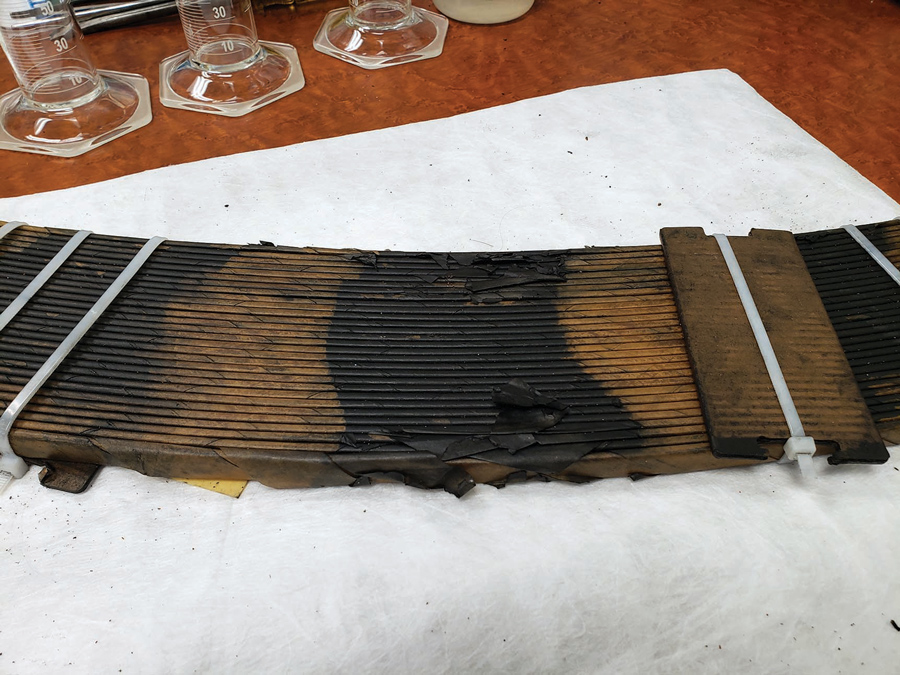
Figure 4: Example of Degraded Insulation
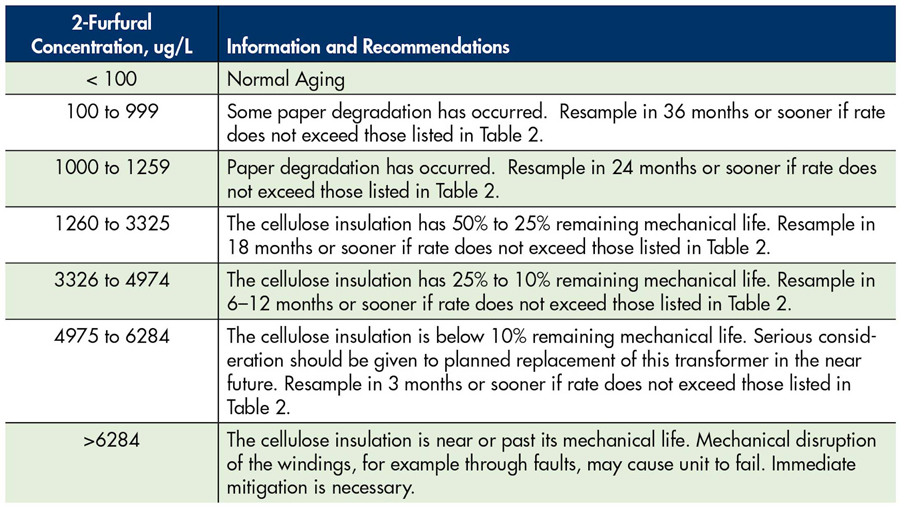
Table 3: 2-Furfural Concentrations
The fate of furanic compounds in the oil can be changed by a number of processes very similar to the fate of acids, water, and gases-in-oil. Vacuum dehydration usually removes about 10% of the furanic compounds. Straight filtration using cellulose filters can remove as much as 15% of the furanic compounds, especially HMF, whereas oil replacement or reconditioning by Fuller’s earth or activated alumina will remove all of the furanic compounds. Removal of furanic compounds does not improve the paper condition, just as degassing does not repair the incipient fault condition of a transformer; it only removes the evidence that the paper degradation has occurred. It is highly recommended to test the insulating liquid for furanic compounds before processing or changeout to serve as a baseline. It usually takes 6–12 months for furanic compounds to migrate from the paper back into the insulating liquid after a processing activity to establish a steady-state condition. Any furanic compounds produced after that point should be added to the baseline value determined before the processing activity to get a more reliable indicator of the true aged condition.
Several other factors can affect the amount of 2-furfural in the insulating liquid. 2-furfural can be decomposed at really high temperatures that produce carbon monoxide and acetylene gases and thus does not adequately indicate that paper degradation has occurred. Additionally, the dicyandiamide used as the thermal upgrading agent in thermally upgraded paper can cause decomposition of 2-furfural through an oxidation reaction and thus yield lower levels than expected. Lastly, because furanic compounds are semi-polar, wet paper tends to bind more strongly to furanic compounds and yields less concentration in the insulating liquid. As a result, another method to determine paper degradation was needed.
METHANOL AND ETHANOL
Because most U.S. and Canadian transformers are built with thermally upgraded paper, the fate of 2-furfural is suspect and may not be the best indicator of paper degradation. In addition, wet paper reduces the concentration of furanic compounds in the insulating liquid. In 2007, research group IREQ (Jalbert et al), which is based in Canada and headed by Dr. Jalbert, was able to determine that the rupture of the glycosidic bond between the cellulose molecules in paper caused the formation of methanol. Additional research by that group developed a method for methanol as an indicator of cellulose aging in 2012. They also found that ethanol was produced but at higher temperatures. That method was developed into ASTM Method D8086, released in 2020.
Research has shown that methanol and ethanol concentrations are not impacted by the wetness of the paper insulation nor the dicyandiamide content of thermally upgraded paper. Additionally, it is an even earlier indicator of paper degradation than furanic compounds. Since the break in the glycosidic bond between two glucose molecule occurs before the glucose molecule is opened up to form the furanic compounds, methanol and ethanol is produced much sooner than furanic compounds. This provides the transformer owner with an earlier alert of a possible issue.
It was also determined that methanol appears to be more thermally stable than furanic compounds; it survives in temperatures about 110°C. Ethanol survives up to 130°C, whereas the temperature range for furanic compounds was 110°C and lower.
The methanol and ethanol concentrations need to be corrected to 20°C to establish a steady-state condition for comparing samples and from which to draw diagnostic value. It should also be noted that transformer design, insulating liquid to paper ratios, and other factors such as the presence of acids may influence the amount of methanol and ethanol produced and dissolved in the insulating liquid.
Table 4 provides information on the methanol concentration and the global calculated DPv value.
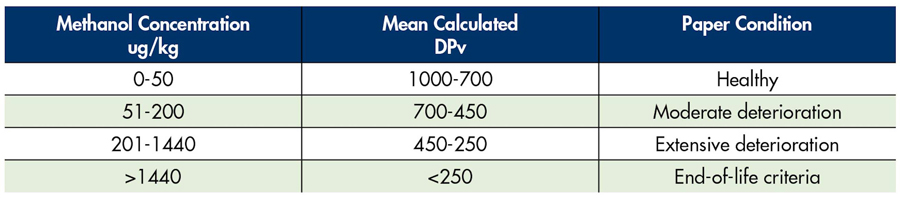
Table 4: DPv Values from Methanol Concentrations
Based on the work by Jalbert et. al, the following interpretation model was presented to calculate the mean DPv value:
DP = DP0/(1.5542(1-e-0.0054 [CH3OH]) + 0.00101[CH3OH] + 1)
where:
DP0 = Starting DPv value after initial factory dry out, usually around 1,000
CH3OH = methanol concentration in ug/kg (ppb)
Methanol and ethanol concentrations suffer the same fate as furanic compounds and other chemical markers from oil processing — most or all is removed even from vacuum treatment alone. However, the migration rate from the paper into the insulating liquid is much quicker than furanic compounds — usually 6 months or less and higher than 89% of the methanol value before treatment. As a comparison, most furanic compounds only return 18% to 26% of the methanol value before treatment.
Currently, analysis and modeling for methanol is for mineral-oil-filled transformers. Hydrolysis reactions that can be found when dealing with natural and synthetic esters may impact the concentrations of methanol and ethanol. Several researchers are currently looking into the degradation mechanism and chemical marker formation.
NOMEX
Since Nomex is a synthetic aramid fiber, no cellulose is present that can degrade. So DPv, furanic compounds, and methanol/ethanol analysis cannot yield any results that would indicate the remaining life of this type of insulation. The only type of analysis that can be performed is tensile strength testing, but this requires going into the transformer to gather Nomex insulation samples for testing; this is not a common practice and is an expensive endeavor. Researchers are actively trying to determine whether some chemical marker can be found in the insulating liquid to aid in determining the condition of the Nomex.
CONCLUSION
Direct tests on paper such as tensile strength and DPv are useful in determining the aging of the paper in the specific locations where the samples were taken. Because water and temperature gradients within the transformer dictate paper aging, degradation is not uniform within the transformer. Therefore, multiple samples are needed to get an adequate assessment. Retrieving the samples is intrusive and costly and thus not often performed.
A more useful and cost-effective approach is to determine the condition of the paper insulation through chemical markers in the insulating liquid. Water/relative saturation, acid content, and dissolved gases-in-oil can be indicators of paper degradation, but cannot be used to determine the remaining life of solid insulation.
Furanic compounds, developed in the late 1980s, are an excellent tool in determining the life of cellulose insulation, especially in free-breathing transformers with Kraft paper insulation. For transformers with thermally upgraded insulation, 2-furfural concentration, which is used to determine an average DPv value, can be compromised by very high temperatures and the dicyandiamide used to thermally upgrade the paper. Because of these factors, methanol and ethanol analysis were developed.
The presence of methanol and ethanol stems from the breakdown of the glycosidic bond between glucose groups in the cellulose molecule. As such, it is an early indicator of paper degradation, and the concentration is not compromised by the presence of dicyandiamide from thermally upgraded paper. In sealed systems with thermally upgraded paper, methanol/ethanol analysis would be the best choice for determining paper degradation. Because methanol/ethanol are light alcohols and volatile, samples must be taken in syringes like DGA in order to make sure the alcohols are not lost to the atmosphere.
For natural- and synthetic-filled transformers, methanol and ethanol production and/or stability may be negatively impacted. All chemical markers in the insulating liquid are negatively impacted by any insulating liquid replacement or processing; thus, baseline values must be established before any processing activity commences.
From the use of indirect tests such as furanic compounds and methanol/ethanol, the amount of paper degradation and an estimate of remaining life can be reasonably ascertained.
REFERENCES
Lewand L.R. and Griffin P.J. “How to Reduce the Rate of Aging in Transformer Insulation,” NETA World, Spring, 1995.
McNutt, W. J. “Insulation Thermal Life Considerations For Transformer Loading Guides,” IEEE Trans. on Power Deliv, Vol. 7, No. 1, Jan. 1992, Pg. 392-401
Lewand, L.R. and Griffin, P.J. “Practical Experience Gained from Furanic Compound Analysis,” Proceedings of the Seventy-Third International Conference of Doble Clients, Boston, MA, 2006
Yoshida, H., Ishioka, Y., Suzuki, T., Yanari, T., Teranishi, T. “Degradation of Insulating Materials of Transformers,” IEEE Trans Dielectr Electr Insul, 1987, EI-22, pg. 795–800
Unsworth, J. and Mitchell, F. “Degradation of Electrical Insulating Paper Monitored with High Performance Liquid Chromatography,” IEEE Trans Dielectr Electr Insul, Vol. 25, No. 4, August 1990, pg. 737-46.
Chendong, X. “Monitoring Paper Insulation Aging by Measuring Furfural Contents in Oil,” 7th International Symposium on High Voltage Engineering, Aug. 26-30, 1991, pp. 139-142.
Jalbert, J., Gilbert. R., Tétreault, P., Morin, B., Lessard-Déziel, D. “Identification of a Chemical indicator of the rupture of 1,4-β-glycosidic bonds of cellulose in an oil-impregnated insulating paper system,” Cellulose, 2007, Vol. 14, pp. 295-309.
Jalbert, J., Gilbert. R., Tétreault, P., Morin, B., and Denos Y. “Kinetics of 1,4-β-glycosidic bonds rupture in cellulose and correlation with methanol formation during ageing of paper/oil systems. Part 1: Standard wood kraft insulation,” Cellulose, 2009, Vol. 16, pp. 327-338.
Jalbert, J., Duchesne, S., Rodriguez-Celis, E., Tetreault, P., and Collin, P. “Robust and Sensitive Analysis of Methanol and Ethanol from Cellulose Degradation in Mineral Oils,” Journal of Chromatography A, Vol. 1256, pp. 240-245, September 21, 2012.
CIGRE. “Field Experience with Transformer Solid Insulation Ageing Markers,” CIGRE Brochure 779, October 2019. Committees A1/D2.
Griffin, P.J., Lewand, L.R., and Pahlavanpour, B. “Paper Degradation By-Products Generated Under Incipient-Fault Conditions,” Minutes of the Sixty-First Annual International Conference of Doble Clients, 1994, Sec. 10-5.
Lance R. Lewand is the Technical Director for the Doble Insulating Materials Laboratory. The Insulating Materials Laboratory is responsible for routine and investigative analyses of liquid and solid dielectrics for electric apparatus. Since joining Doble in 1992, Lance has published over 75 technical papers pertaining to testing and sampling of electrical insulating materials and laboratory diagnostics. He is actively involved in professional organizations including the American Chemical Society; has served on the ASTM D-27 since 1989 and chairs ASTM Committee D-27 as well as Subcommittee 06 on Chemical Tests; is secretary of the Doble Committee on Insulating Materials, and represents the U.S. on the National Committee for TC10 of the International Electrotechnical Commission (IEC) and ISO TC28. Lance is a recipient of the ASTM Award of Merit for Committee D-27. He received his BS from St. Mary’s College of Maryland.
David Koehler is the Business Development Manager, Professional Services for Doble Engineering Company. He has 23 years of experience in the testing of insulating liquids and management of analytical laboratories. He has provided numerous technical presentations and published technical articles within the power industry and is an active contributor at NETA’s PowerTest Conference. David is Vice President-elect for IEEE Member and Geographic Activities (MGA) and a member of IEEE’s Honor Society, HKN. He served on the IEEE Board of Directors from 2019–2020 and will serve on the IEEE Board of Directors again in 2022. David is a member of the ASTM D-27 Technical Committee on Electrical Insulating Liquids and Gases and serves as an Advisory Board Member for Engineering and Technology at Embry-Riddle Aeronautical University, Worldwide Campus. David is a past Executive Committee member of the Indiana American Chemical Society. He received a BS in chemistry from Indiana University and obtained his MBA.